Laser Processes Promise Better Artificial Joints, Arterial Stents
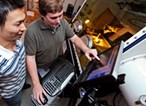
Researchers are developing technologies that use lasers to create arterial stents and longer-lasting medical implants that could be manufactured 10 times faster and also less expensively than is now possible.
New technologies will be needed to meet the huge global market for artificial hips and knees, said Yung Shin, a professor of mechanical engineering and director of Purdue's Center for Laser-Based Manufacturing.
The worldwide population of people younger than 40 who receive hip implants is expected to be 40 million annually by 2010 and double to 80 million by 2030. In addition to speeding production to meet the anticipated demand, Shin said another goal is to create implants that last longer than today's.
"We have 200,000 total hip replacements in the United States," he said. "They last about 10 years on average. That means if you receive an implant at 40, you may need to have it replaced three or four times in your lifetime."
One of the researchers' techniques works by depositing layers of a powdered mixture of metal and ceramic materials, melting the powder with a laser and then immediately solidifying each layer to form parts. Because the technique enables parts to be formed one layer at a time, it is ideal for coating titanium implants with ceramic materials that mimic the characteristics of natural bone, Shin said.
Findings will be detailed in a presentation this week during the International Medical Device Expo's Advanced Laser Applications Conference in San Jose, Calif.
"Titanium and other metals do not match either the stiffness or the nature of bones, so you have to coat it with something that does," Shin said. "However, if you deposit ceramic on metal, you don't want there to be an abrupt change of materials because that causes differences in thermal expansion and chemical composition, which results in cracks. One way to correct this is to change the composition gradually so you don't have a sharp boundary."
The gradual layering approach is called a "functionally gradient coating."
Researchers used their laser deposition processes to create a porous titanium-based surface and also a calcium phosphate outer surface, both designed to better match the stiffness of bone than conventional implants.
The laser deposition process enables researchers to make parts with complex shapes that are customized for the patient.
"Medical imaging scans could just be sent to the laboratory, where the laser deposition would create the part from the images," Shin said. "Instead of taking 30 days like it does now because you have to make a mold first, we could do it in three days. You reduce both the cost and production time."
The laser deposition technique lends itself to the requirement that each implant be designed specifically for each patient.
"These are not like automotive parts," Shin said. "You can't make a million that are all the same."
The process creates a strong bond between the material being deposited and the underlying titanium, steel or chromium. Tests showed the bond was at least seven times as strong as industry standards require, he said.
The researchers use computational modeling to simulate, study and optimize the processes.
Additional research is needed before the techniques are ready for commercialization. Future work will involve studying "shape-memory" materials that are similar to bone and also have a self-healing capability for longer-lasting implants.
The researchers also are developing a technique that uses an "ultra short pulse laser" to create arterial stents, which are metal scaffolds inserted into arteries to keep them open after surgeries to treat clogs. The laser pulses last only a matter of picoseconds, or quadrillionths of a second.
Because the pulses are so fleeting, the laser does not cause heat damage to the foil-thin stainless steel and titanium material used to make the stents. The laser removes material in precise patterns in a process called "cold ablation," which turns solids into a plasma. The patterns enable the stents to expand properly after being inserted into a blood vessel.
The work is funded by the National Science Foundation.
SOURCE: Purdue University